


Fourier transform microwave (FTMW) spectroscopy can provide accurate and detailed information about the structure and the dynamics of van der Waals complexes. The complexes are produced in a pulsed supersonic jet expansion of a gas mixture into a vacuum chamber. The temperature of the sample in the jet expansion is on the order of a few Kelvin, and the complexes are stabilised in this nearly collision-free environment. Interaction with a pulse of microwave radiation causes the dipole moments of the complexes to align, resulting in a macroscopic polarization of the 'ensemble' of complexes. After the pulse, relaxation occurs, and the decay of this polarization with time (free induction decay or FID) is recorded. This principle might be clarified in the following Flash movie:
|
|
The principle of FTMW spectroscopy is thus very similar to that of pulsed nuclear magnetic resonace (NMR) spectroscopy which is also based on the excitation of a molecular ensemble with a pulse of coherent radiation and the subsequent detection of the molecular emission signal.
To obtain a spectrum (as a function of frequency), a mathematical operation called a Fourier transform is applied to the time domain signal:
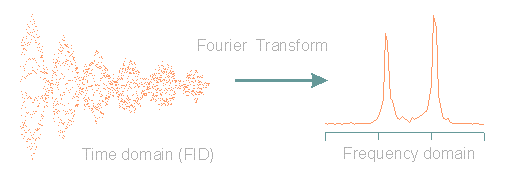
The analysis of the resulting spectra results in the determination of rotational constants which are related to the principal moments of inertia of the complex. From these constants, the molecular geometry of the complex can be determined.
The sample cell of our FTMW spectrometer is a microwave cavity that consists of two spherical aluminum mirrors situated in a vacuum chamber. The sample enters the chamber via a pulsed nozzle near the center of one mirror. The microwave radiation enters and exits the chamber through a wire hook antenna at the center of the same mirror. The distance between the mirrors is adjusted to a “resonance” position that depends on the frequency of the microwave radiation.
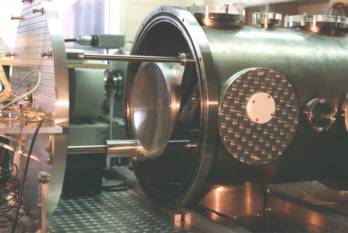
This experimental setup makes it possible to measure rotational transitions in the frequency range between 4 and 26 GHz with line widths of approximately 7 kHz and a frequency accuracy of 1 kHz.

Back to Research

|